The Amazing Possibilities of Neuroplasticity and Neurogenesis
You may have heard the phrase “neurons that fire together wire together.” This short phrase summarizes the synaptic plasticity theory of learning described by Canadian psychologist Donald Hebb in his 1949 book The Organization of Behavior.
Hebb explained how the connections between neurons (brain cells) change as a result of repetitive firing. So when you repeat a movement like swinging a golf club over and over, the neural pathways involved in controlling that movement become stronger and faster. Not only do existing synapses (junctions between neurons) begin to fire more efficiently, but new synapses are formed and other neurons are recruited to get in on the action. As a result, your golf swing becomes more automatic, reliable, and forceful the more often you practice.
That is neuroplasticity: your brain’s ability to change and adapt based on input and use. The concept of neuroplasticity had been previously proposed by others, most notably American psychologists William James and Karl Lashley, and Polish neuroscientist Jerzy Konorski, but it was largely ignored by the scientific community until Hebb brought the concept to the forefront in his groundbreaking book.
In 1965, scientists took another huge step forward in their understanding of the brain when a study demonstrated that new neurons are produced in the brains of adult rats. This was the first proof that neurogenesis, the process by which new neurons are formed in the brain, occurred after birth. Up until that point, scientists believed that higher vertebrates (reptiles, birds, and mammals) were born with all the neurons they were going to have for their entire lives, and that neurons gradually died over time and never got replaced.
But was the same true for human brains? In 1998, researchers showed that adult human brains produced new neurons throughout life as well—and the brain-fitness industry boomed.
Research on neuroplasticity and neurogenesis is still in its infancy. Understanding how these processes work pose incredible opportunities for preventing and recovering from neurodegenerative diseases, improving quality of life as we age, and reaching our human potential. In this post I’ll talk about how new neurons are produced in the brain, how the brain adapts to use, and what you can do to increase—and decrease—neurogenesis.
How new neurons are born—and what happens next
Neural stem cells and progenitor cells give rise to immature neurons called neuroblasts. These newborn brain cells can then stay where they are or migrate, and then mature and integrate into our neural circuitry.
The hippocampus, which plays an important role in memory and spatial navigation, is one of the brain areas where neurogenesis has been observed in humans. In Alzheimer’s disease, the rate of neurogenesis in the hippocampus progressively declines as the disease advances. Scientists have found that enhancing neurogenesis in rodents improves hippocampal size and function, and decreases symptoms of Alzheimer’s disease. Their findings suggest that stimulating neurogenesis in humans, through environmental enrichment, running, and other proven methods, may be a key therapeutic approach in preventing and reversing Alzheimer’s in humans. The size of the hippocampus in people with major depressive disorder is significantly reduced, suggesting that a similar approach could be used for treating depression.
Another part of the brain where neurogenesis occurs is the subventricular zone (SVZ) of the lateral ventricals. Neuroblasts produced in the SVZ migrate through the rostral migratory stream (RMS) to the olfactory bulb, which is involved in our sense of smell.
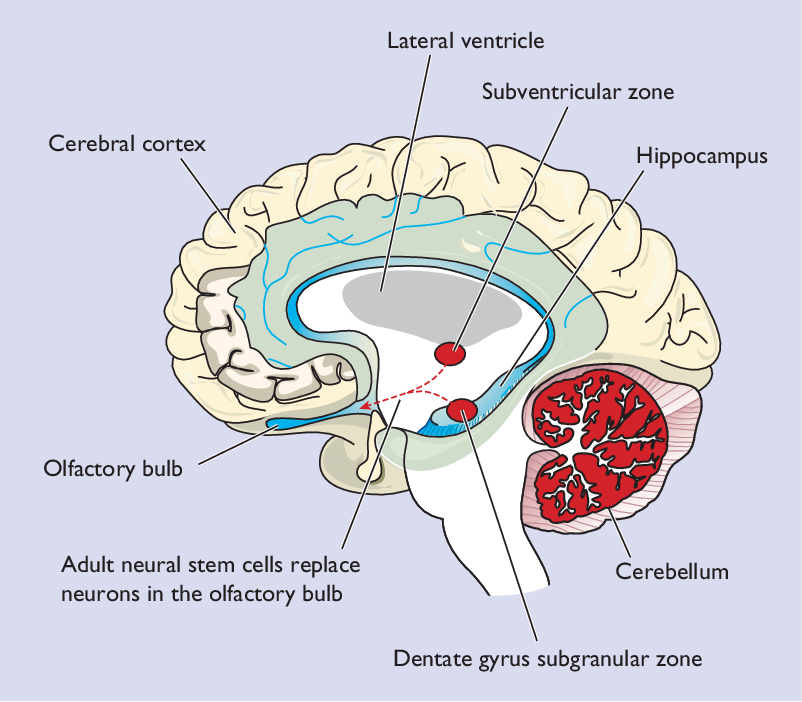
Research shows shows neurogenesis occurring in the cerebral cortex of adult mice, and one study of stroke sufferers shows evidence of new neurons in the human cerebral cortex (the outer layer of the brain that plays an important role in attention, perception, awareness, thought, memory, language, and consciousness). There are also cells that express stem cell markers in the adult cerebellum, which is responsible for coordinating movement.
And in 2003, scientists were surprised to find that neurogenesis occurs in the substantia nigra, the brain area affected in Parkinson’s disease. In 2016, researchers found that neural progenitor cells in the brains of adult mice replenish the dopaminergic neurons that are lost in Parkinson’s disease. The researchers suggest that neuron loss in Parkinson’s disease may result from inhibition of neurogenesis. They note that neurogenesis has been difficult to prove due to limitations of current cell lineage tracing methods, and they were able to demonstrate neurogenesis of nigral neurons using a new tracing model that they developed. Neurogenesis of nigral neurons is not limited to mice—viable neural stem cells have been found in the brains of people with Parkinson’s as well.
As research on neurogenesis continues, scientists will discover more exciting things about how and where in the brain new neurons are produced. But producing new neurons is only the first piece of the puzzle. Can neuronal migration—the movement of neuroblasts—occur throughout the brain, based on where we need new neurons? Research is only beginning to answer this question, but so far it looks like the answer is yes.
“Neuroblasts derived from the ventricular‐subventricular zone (V‐SVZ) are a special population that can migrate over long distances in the adult brain; this is an important characteristic for providing new neurons for neuronal regeneration to areas that are distant from the germinal zone, especially in large primate brains. The long‐distance directional migration of these neurons is controlled by various endogenous and exogenous factors.”
–Mechanisms of neuronal migration in the adult brain. March 2017.
For example, after a brain injury, neuroblasts produced in the V-SVZ migrate toward the injury to repopulate the injured brain tissue. The neuroblasts are guided toward the injury by chemoattractants and structures including blood vessels and neuroblast chains. The immature neurons tend to change direction and explore their environment more than those that stay within the well-traveled rostral migratory stream (RMS). They’re forging new pathways through the brain, so their migration is inefficient. When they do arrive at the site of the injury, they mature into functional neurons.
A 2017 study by researchers at University of Alabama at Birmingham showed something else that happens after new neurons are born. Neuroblasts produced in the hippocampal dentate gyrus synapse with existing neurons in the cerebral cortex, taking the place of older neurons in the neural circuitry. So instead of creating additional synapses, neuroblasts replace and weed out less-fit neurons, which then die. This finding shows the role that new neurons play in “neural pruning,” the process by which neurons that aren’t used on a regular basis are eliminated in order to increase the efficiency of regularly-used circuits.
Getting your neurons where they need to go: Activity-dependent neuroplasticity and neural migration
Activity-dependent neuroplasticity is what happens as existing neurons form new synapses and create new circuits based on how we choose to use our brains; this is learning. Your brain is constantly adapting to how it’s being used. If you practice the tango every day, the neural pathways involved in controlling your dance moves will continually get stronger and more efficient. The secret to using activity-dependent neuroplasticity in your favor is to learn your new skill and then practice, practice, practice.
Neurologists in Germany did brain scans of people as they learned to juggle and compared them with controls. The scans clearly showed how brain areas involved in processing and storing complex visual motion increased in size over three months as the volunteers learned to juggle. Then three months later, after discontinuing the juggling practice, these brain areas had decreased in size, losing about half of the gray matter they had gained by juggling.
Similar cortical reorganization occurs in people and animals who have lost limbs due to amputation. After amputation, people and animals adapt by increasing use of their intact limbs; for example, someone who has lost their right hand will start using their left hand much more than they used to. Parts of the motor and somatosensory cortices that control and sense the intact body parts adapt to increased use by growing in size, while the parts that control and sense the lost limb decrease in size or sometimes move into nearby brain areas due to lack of use.
Now scientists are finding that if you stimulate a certain area of the brain, it causes neurons to migrate toward that area; this is activity-dependent neural migration.
In 2009, researchers in South Korea showed how hippocampal neurons migrate toward a site of electrical stimulation. Neurons communicate with each other via electrical signals called action potentials and chemical signals called neurotransmitters. When we’re using a part of our brain, electrical activity in that area increases, and this experiment clearly demonstrated how increasing electrical activity attracts new neurons. Similar results have been found using cortical neurons.
Are you ready to make the most of your neuroplasticity and neurogenesis? First, you need to stop doing things that inhibit neurogenesis. Then you need to start doing things—or keep doing them!—that promote neurogenesis. Finally, you need to stimulate neuroplasticity and neurogenesis in the brain areas where you want them to occur, by doing the activities you want to get better at.
What gets in the way of us producing new neurons?
Four of the lifestyle factors that inhibit neurogenesis the most are are stress, alcohol consumption, sleep deprivation, and diet.
Chronic stress decreases neurogenesis in the hippocampus, and also lowers the chances that the new neurons that do get produced will survive. Neuroinflammation (the inflammatory response of the immune system when it occurs in the brain) that results from stress also likely prevents the production of new neurons. And sadly, stress experienced in childhood can inhibit neurogenesis in adulthood. Constant stimulation and worrying about the future is not good for brain health—we need to slow down and relax our minds in the same way we rest our bodies.
Regular alcohol consumption reduces the size of the hippocampus to a degree proportional to the amount that people drink. The same is true for overall brain atrophy; researchers found that brain volume decreases in proportion to alcohol consumed, and the effect is measurable even in light and moderate drinkers in comparison to non-drinkers. Luckily, it seems that the effects can be reversed. While heavy alcohol consumption inhibits neurogenesis, subsequent abstinence allows neurogenesis to return to relatively normal levels in a short period of time.
Both sleep deprivation and sleep fragmentation (disrupted sleep) inhibit neurogenesis in the hippocampus. Even a single day of sleep deprivation reduces the rate at which new neural cells are produced. Not to worry—normal rates of neurogenesis can be recovered within about two weeks after adequate sleep is resumed.
Researchers have found a number of dietary factors that prevent the production of new neurons. It probably won’t come as a surprise that eating a high-fat diet is one, and consuming refined sugars is another. Being deficient in vitamin A and vitamin B can also inhibit neurogenesis. And pay attention to the texture of your food: eating a diet of soft foods decreases neurogenesis, while eating solid foods that require more chewing increases production of new neurons.
Seven ways to stimulate neurogenesis
Exercise is one of the best ways to increase neurogenesis, largely because it boosts production of brain-derived neurotrophic factor (BDNF). BDNF is a protein that acts like Miracle-Gro for brain cells: it stimulates the growth of neuroblasts, helps them survive, and encourages the formation of new synapses. The positive effects of exercise are enhanced by environmental enrichment (EE), which can include being in new, stimulating surroundings or going outdoors.
Sustained aerobic exercise like running increases neurogenesis, while resistance exercise has not been shown to have the same effect. Dr. John Ratey, the author of Spark: The Revolutionary New Science of Exercise and the Brain, recommends doing both aerobic exercise and activities that demand focus and coordination, like martial arts, dance, rock climbing, and yoga, in order to fully stimulate your brain.
Learning improves the chances that neuroblasts will survive, mature, and integrate into neural circuitry. This is why continuing to stimulate your brain by learning new things throughout life is so important. For best results, scientists recommend exercising first in order to increase the production of new neurons, then spending time learning your new skill to help the new neurons survive and integrate. And be sure to let your learning be fun; when learning becomes stressful, it can decrease neurogenesis.
In rat experiments, sex increases the number of new neurons in the hippocampus. But regular sexual activity is best; a single sexual encounter also increases levels of the stress hormone corticosterone. Daily sexual encounters for 14 consecutive days do not raise corticosterone levels, and actually decrease anxiety behavior while still promoting neurogenesis. It’s also interesting to note that female rats only experience an increase in neuronal survival when they’re in control of the sexual encounter. Researchers speculate that when they’re not in control, female rats experience stress which prevents their new neurons from surviving. Neither of these experiments have been replicated in humans, but it’s easy to see how the same principles apply to short-term versus long-term relationships and male-female dynamics.
Living in groups helps us survive, so it’s not surprising that socializing promotes production of new brain cells. Neurogenesis is higher in socialized rats compared to rats kept in isolation. Isolated rats show a significant reduction in BDNF in the hippocampus, and they also perform worse on memory tasks. Social isolation also increases stress, and even cancels out the positive effects of running on neurogenesis. So if you live alone, be sure to make socializing part of your regular routine.
There is a long list of foods that promote neurogenesis. You may have heard that blueberries are brain food, and it turns out that strawberries are too (it’s the polyphenols). Grapeseed extract, turmeric, green tea, and resveratrol (found in peanuts, tree nuts, grapes, cocoa, wine, and berry fruits) also promote the growth of new neurons. And fatty fish, seaweed, algae, walnuts, and flax, chia, and hemp seeds contain omega-3 polyunsaturated fatty acids (PUFAs), which have been shown to promote hippocampal neurogenesis and improve spatial memory.
The idea of fasting is not a popular one—most people don’t like being hungry. But the practice of intermittent fasting is a new trend due to research showing its benefits for a wide range of health conditions. We evolved to survive during periods of fasting because food wasn’t always available. As a result, intermittent fasting is built into our physiology, and research shows that it benefits our health in many ways. Intermittent fasting lowers our insulin level and blood pressure, reduces inflammation, helps clear out toxins and damaged cells, improves our stress resistance, lengthens our lifespan, and reduces incidence of diabetes, obesity, and cancer. And of course, it increases neurogenesis and levels of BDNF, and prevents neuron death in the hippocampus.
The simplest form of intermittent fasting limits all food intake to a certain window of time, like 6 hours a day. Researchers recommend the circadian rhythm fasting approach, in which you start eating in the morning and finish consuming all your food by mid-afternoon or early evening. A 2018 study of men with prediabetes clearly showed the positive effects of early time-restricted feeding (eTRF): lower insulin levels, improved insulin sensitivity, and lower blood pressure and oxidative stress. Surprisingly, the eTRF group also had less desire to eat in the evening. Eating a big meal in the evening is probably not good for our health, and researchers suggest that the ways our circadian rhythms affect our body temperature, biochemical reactions, hormone levels, physical activity, and digestion of food may be why.
Finally, you can stimulate neurogenesis with meditation. The cerebral cortex of people who practice meditation is thicker in the areas associated with attention, interoception (internal sense of your body), and sensory processing. Differences in cortical thickness correlates with meditation experience, and meditation may offset age-related cortical thinning. Experienced meditators also have larger hippocampi than non-meditators, and yoga and meditation increase levels of BDNF.
Why it can be hard to wrap your mind around neuroplasticity and neurogenesis
Everyone understands “brain training” on a practical level—this is why we practice skills over and over to get better at them. We know that learning and repetition work. It’s another thing entirely to embrace neuroplasticity and neurogenesis as physiological processes that you can control and take action toward improving. Measuring changes in your brain function is tough because you can’t see them in the same way you can see changes in your body or track improvements in your physical performance.
It can also be easy to think you’re imagining things. It’s normal for your mood, energy, focus, cognitive abilities, and stress level to fluctuate from day to day. How do you know if something different represents a structural and functional change in your brain?
When you make a lifestyle change—like getting more sleep, reducing alcohol intake, starting to meditate, or exercising more—notice the effects. Do you feel happier or less stressed out? Are you able to focus better at work? Notice how you feel every day as you continue your new habit, and you’ll start to see trends. When a trend persists over time, you can feel confident that you’re making a lasting change in your brain.
And try envisioning the changes taking place in your brain. When you get faster at your computer game, visualize the neural circuits that control your play efficiently lighting up and sending their messages. When you find yourself reacting less to potentially stressful events, imagine the calmness in your brain as it decides to not activate your stress response.
We have just as much control over the health and function of our brains as we do our bodies. But because the science is fairly new and changes in the brain are harder for us to measure, we haven’t quite mastered how best to train our brains and maintain their health throughout our lives. For now, it may take a little faith along with a lot of self-awareness to keep your brain in the best possible shape.
Recommended reading:
The Pain Relief Secret: How to Retrain Your Nervous System, Heal Your Body, and Overcome Chronic Pain by Sarah Warren, CSE
Somatics: Reawakening the Mind’s Control of Movement, Flexibility and Health by Thomas Hanna